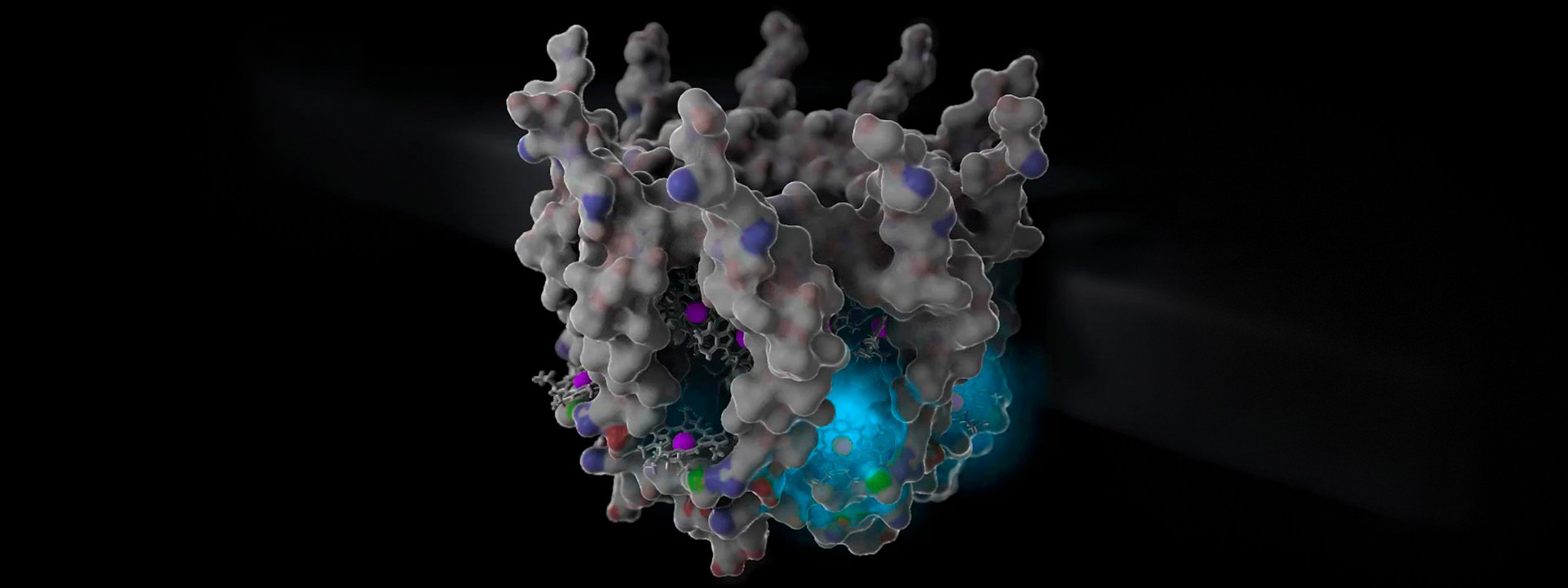
ENERGY FLOW IN PLANTS
The manner in which photosynthetic plants and bacteria harvest individual photons from the sun has been an open question for some time. They manage to capture most of the energy that arrives, and then transport it over long distances without much loss. In this work, we set out to visualize how energy flows immediately after arriving at a photosynthetic energy harvesting complex. An important part of this work was to represent in full physical detail exactly how the atoms within the system vibrate.
The difficulty one faces in studying a photosynthetic molecular complex is its size. For example, the molecule shown in this video (called “LH2”) has lots of atoms and lots of electrons. And so a full representation of how everything moves is a non-trivial challenge. To do it, we built a multi-tiered parallel computational framework for calculating atomic cartesian gradients on each and every excited state. We also introduced some approximations for how to treat cartesian gradients of the excited state dipole moments and the transition dipole moments. We were then able to run surface-hopping simulations to treat the explicit dynamics of both the atoms and the electrons.
The picture of energy flow which emerges is shown in the movie. The key thing which this movie highlights (compared to previous work) is the fact that the atomic motion is explicitly accounted for, along with the electronic motion (which is shown as diffuse blue clouds). You need to look closely at the video to see the atomic motion, because your eyes are more attuned to the flashing blue electronic motion compared to the subtleties of the vibrational motion. But it’s definitely all in there if you look closely!
The picture of energy flow which emerges from this video is one where the excited states fluctuate rapidly as a result of the underlying vibrational dynamics of the atoms. The excited electronic states are delocalized over multiple chromophores and undergo frequent crossing on a femtosecond timescale. Every crossing offers an opportunity to transfer amplitude from one excited state to another. The result is a sort of highly connected excited state network: the frequent crossings create scenario where the states are in a sort of constant “communication” with one another, allowing excitation localized in any one state to travel far and fast.
PUBLICATIONS
A. Sisto, C. Stross, M. van der Kamp, M. O’Connor, S. McIntosh-Smith, G. T. Johnson, E. G. Hohenstein, F. R. Manby, T. J. Martinez, D. R. Glowacki, “Atomistic absorption spectra and non-adiabatic dynamics of the LH2 complex with a GPU-accelerated ab initio exciton model”, Physical Chemistry Chemical Physics (2017), 19, 14924